








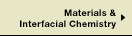














|
|


Overview
Theory, Modeling, and Simulation on Multiple Scales
From electronic structure and molecular interactions,
through statistical-physical theory,
towards modeling of system functioning
The Theory and Modeling Group at NINT has been developing theory, modeling, and simulation of nanosystems on multiple length and time scales. Our approach integrates the following levels, from the electronic structure to system functioning:
1) Electronic properties (Kohn-Sham Density Functional Theory)
2) Molecular simulations (Monte Carlo, Molecular Dynamics)
3) Molecular solvation (Integral Equation Theory of Molecular Liquids)
4) System functioning (Continuum Kinetic Theory and Kinetic Monte Carlo).
Integral Equation Theory of Molecular Liquids (3) is a statistical physical theory that bridges the gap between the atomistic simulations (2) and the system functioning level (4). The integral equation theory takes proper account of molecular interactions in condensed matter systems, such as molecular liquids and solutions, complex fluids, polymers in melts and solutions, biomolecules and supramolecules in solution, solid solutions, defects in crystals, and disordered materials.
A challenge of nanotechnology is that the essential properties of nanosystems are determined by molecular interactions, whereas the overall length and time scales are usually very large. For instance, the relaxation time in liquid crystals can vary from microseconds to minutes, depending on the environment. Our bottom-up approach enables efficient simulation of nanosystems at realistic length and time scales, while staying on ab-initio grounds.
Approach
We use integral equation theory to provide realistic physical and chemical description of solutions, complex liquids, and other disordered systems, including nanoporous materials, solid solutions, and defects in crystals. This approach employs atomistic force fields, such as the Optimized Potential for Liquid Simulation (OPLS) or Assisted Model Building with Energy Refinement (AMBER). By solving integro-differential equations for the correlation functions in condensed matter, the formalism yields the structure of the system, statistically averaged over the ensemble of all possible configurations. The thermodynamic functions (such as the free energy, entropy, compressibility, partial molar volume, etc.) are in turn derived analytically from the correlation functions. The method properly accounts for chemical specificities of the species involved.
A crucial advantage of the integral equation approach is that it samples the phase space of the system on arbitrarily large volume and time scales. This overcomes the well-known limitations of molecular simulations which treat individual trajectories of a finite number of particles in a finite, quite limited time frame.
In the three-dimensional form, this theory provides a detailed solvation structure of molecules and supramolecular clusters in solution and at interfaces.
We self-consistently integrate the statistical physical theory with the other levels in our bottom-up approach. This yields computationally efficient simulation of nanosystems.
Examples:
SCF Kohn-Sham DFT / 3D-RISM-KH
RISM-KH / Kinetic MC
Research Interests
- Solid surfaces and interfaces
- Synthesis of nanocrystals (PVD, CVD, and electrochemistry)
- Particle-surface interactions
- Reactions at solid-liquid interfaces
- Nanoheterogeneous catalysis
- Nanoporous materials for sorption and electrochemical devices
- Electronic structure of quasi-particles at semiconductor surfaces
- Photonics
- Theory of molecular solvation
- Soft matter nanostructures in complex fluids and solutions
- Block copolymers in melts, solutions, and at surfaces
- Phase separation, microemulsions and suspensions
- Colloids in complex liquids and solutions
- Volumetric properties of organic and biomolecules
- Supramolecular architectures in solution
- Supramolecular self-assembly and conformational stability of synthetic organic and biomolecules
- Molecular recognition
- Bioadsorption
- Dynamics and kinetics of protein interactions
|