Newsletter for the Canadian Antarctic Research Network - Vol 31, November 2012
Download this newsletter in PDF format [1.34 MB]
Contents
Canada Research Chair for Christian Haas
Ionospheric GPS Scintillation Monitoring in the Arctic and Antarctic
XXXII SCAR Meetings and Open Science Conference, Portland, Oregon, U.S.A.,13–25 July, 2012
XXXII SCAR Meeting Delegate Committee on Scientific Affairs
Ecology of sub-Antarctic Islands: an Eco-System Approach for Understanding Population Dynamics and their Drivers
Leigh J. Gurney and Evgeny A. Pakhomov
Islands have long been considered important for biological research because their isolation provides a unique platform from which ecological theory can be derived and tested. One of the best known research endeavours led to Darwin’s theory of evolution, aided by insights gained from his studies of the flora and fauna of the Galápagos Islands. Less well known is that the subantarctic islands were identified by Darwin, in a letter to Lyell, as being potentially important in furthering the understanding of natural history (http://www.darwinproject.ac.uk/
entry-2050):
But if there be any choice or power in the scientific men to influence the places, this wd. be very desirable; it is my most deliberate conviction that nothing would aid more Natural History, than careful collecting & investigating all the productions of the most insulated islands, especially of the southern hemisphere.…
– Letter from Charles Darwin to Charles Lyell, 11 February 1857
The isolation of such islands provides an opportunity to study an area where anthropogenic effects are limited and/or known. Nature is complex, and forming an understanding of a system in its natural state is vital. Once the current and past state of a system is understood, it is then possible to test future scenarios by including variations of exploitation and environmental drivers.
The Prince Edward Islands (PEIs) are an isolated Southern Hemisphere archipelago consisting of two small volcanic islands (Marion and Prince Edward). They lie in the subantarctic Indian Ocean, about halfway between the African and Antarctic continents. Although small in area, 290 and 45 km2 respectively, the islands are host to large populations of seasonal seabirds and seals that use them as a refuge for breeding and moulting, and as a base from which they forage, many travelling great distances to feed. Satellite tracking of seasonal residents has shown that feeding is focused on frontal features, both to the north and south, as well as mesoscale eddies generated both up and downstream of the islands. Foraging ranges vary between species with some travelling as far afield as the ice edge of Antarctica, while others limit themselves to nearshore waters.

Figure 1 Elephant seal. Photo: Genevieve Jones
Scientific observations began here following the annex ation of the islands by South Africa in the late 1940s. Weather observations at a meteorological station were later expanded to include the collection of biological data. Population surveys of many of the land-based top predators have been completed regularly since the 1970s so an extensive time series of data now exists for the islands. Disparate population trends have been observed for many of the top predators in the system, including the seals, penguins, albatross and the sole target of an ongoing fishery, the Patagonian toothfish.
Seals
Elephant seals (Fig.1) and fur seals are both found here. The population of elephant seals (Mirounga leonina) has declined by more than 60% since monitoring began in the 1950s (Bester and Hofmeyr, 2005). The reasons behind this decline are uncertain though many hypotheses have been put forward, including competition with fisheries, intraspecific competition, as well as climate change. In contrast, the fur seal population (the more numerous Arctocephalus tropicalis and less common Arctocephalus gazella) has recovered to what is thought to be pre-exploitation levels. Increasing from a few hundred individuals in the early surveys in the 1950s to ~150,000 today, the fur seals now dominate the PEI seal biomass (Hofmeyr and others, 2006).
Penguins
Penguins are the largest contributors to biomass of the land-based top predators (seals and seabirds) and are made up of four species which vary in size, diet, and foraging range and exhibit different population trends (Adams and others, 1985; Adams, 1987; Adams and Wilson, 1987; Brown, 1987; Crawford and others, 2003a, b, c). The king penguins (Fig.2; Aptenodytes patagonicus), having a predominantly small pelagic fish diet, are the largest and forage the furthest. Population estimates, though few data exist, suggest that there has been little change in the number of this species at the PEIs. The gentoo penguin (Fig.3; Pygoscelis papua), second largest in size and also predominantly a fish eater, though restricted in the distance that it forages (<40 km from shore), declined through the 1990s, but has shown recent recovery. The two smallest penguins, the macaroni (Fig.4; Eudyptes chrysolophus) and southern rockhopper (Fig. 5; Eudyptes chrysocome filholi), are summer breeders, are intermediary in their foraging range (100–300 km from shore), and have been in recent decline. Both have a predominantly crustacean diet. The rockhoppers, in particular, declined by 66% between 1994/5 and
2002/3 (Crawford and others, 2003c). Various hypothe-ses have been put forward to explain these disparate trends. In terms of conservation status, according to Birdlife International (www. birdlife. org, downloaded 27/09/2011), the gentoo penguin is classified as ‘Near Threatened’, both the macaroni and southern rockhopper penguins as ‘Vulnerable’, while the king penguins are of ‘Least Concern’.

Figure 2 King penguins. Photo: Genevieve Jones.

Figure 3 Gentoo penguins. Photo: Genevieve Jones
The king penguins at PEI account for approximately 13% of the worldwide population, with the other three penguin groups making relatively small contributions to the world’s population (macaroni 4%, southern rockhopper 5% and gentoo 0.5%).

Figure 4 Macaroni Penguin colony. Photo: Genevieve Jones.

Figure 5: Southern rockhopper penguins. Photo: Genevieve Jones.
Albatross
Five albatross species are considered resident at the islands: the wandering (Diomedea exulans), grey-headed (Thalassarche chrysostoma), Indian yellow-nosed (T. carteri) and lightand dark-mantled sooty (Phoebetria palpebrata and P. fusca). Together, the albatross populations account for a small percentage of the avian biomass (<3%), but they are considered important in terms of their conservation status. Birdlife International classifies both the wandering and grey-headed albatross as ‘Vulnerable’, the Indian yellow-nosed and dark-mantled sooty as ’Endangered’, while the light-mantled sooty is ‘Near Threatened’. Population dynamics of albatross species (e.g., wandering and greyheaded) have been monitored with long-term trends observed (Nel and others, 2002a) showing declines in the early 2000s which have been linked to the increase in tuna long-lining as well as large-scale Illegal Unregulated and Unreported (IUU) long-line fishing for Patagonian toothfish (Nel and others, 2002b, c). However, mitigation measures have reduced the by-catch of birds significantly (CCAMLR, 2010). Patagonian Toothfish The Patagonian toothfish (Dissostichus eleginoides) is the sole target of the ongoing fishery at the PEIs. This Southern Ocean resource was discovered in the 1970s and 1980s and exploitation at the PEIs is believed to have started in the early 1990s. Large IUU catch early on in the fishery (estimated at ~20,000 tons for the 1996/97 season [Brandão and others, 2002]) resulted in its collapse. Today, a licensed fishery is ongoing and recent data show some rebuilding of the stocks in the vicinity of the islands (CCAMLR, 2011).
Such population fluctuations highlight concerns which include both exploitation and conservation issues. In 2005, work began at the University of British Columbia (UBC) on a model of the PEIs to assess the ecosystem as a whole, to investigate the effects of known drivers, and to provide scenarios on the potential effects of environmental change. A combination of: a) a rich published dataset from this South African territory, b) the expertise of the “Laboratory of Zooplankton and Micronekton Ecology” (UBC), led by Dr Evgeny Pakhomov, with more than 15 years fieldwork at the study site; and c) the modelling tool Ecopath with Ecosim (EwE), developed at the UBC Fisheries Centre, enabled this development. The model incorporated over40 years of oceanographic and biological data for parameterization of the system. Remotely sensed, as well as in situ, oceanographic data were used for primary producer estimates. Biological datasets, that included population censuses for biomass estimates, estimates of production and consumption for all biological components, trophic pathways (informed from stomach contents and stable-isotope signatures), and fisheries information, were all combined for the model construction.
In developing the model, it has been possible to highlight data gaps and areas for future research. The lack of information on, and the relative importance of, the nekton groups in particular have been identified as requiring further attention. The model provides a platform from which to investigate all drivers and the direct and indirect effects of them on the system as a whole and on individual groups. The more holistic approach to marine-resource management by using ecosystem models, capable of capturing the dynamics of the system, can provide useful insights to past and future scenarios, taking both fisheries and conservation concerns into account, and aid in the management of such systems. In the case of the exploitation of fur seals and Patagonian toothfish, it has been possible to reconstruct the population dynamics through fishery drivers. Although the fisheries have now been limited, they did impact the system heavily, removing thousands of tons of production while in operation. In the case of the fur seals, the effects of exploitation on the ecosystem as a whole lasted over a century. With respect to the Patagonian toothfish, results from the model agree with single-species assessments, aligned with pre-exploitation biomass estimates, of the ‘less-pessimistic’ scenario suggested by Brandão and Butterworth (2009; Brandão and others, 2002) and the rebuilding of stocks evident in recent years.
Now that the model has been shown to be capable of reproducing observed population changes, it is hoped it can be used as a tool to explore alternative drivers (environmental) and to identify and develop relevant ecosystem indicators to help move marine-resource management to an ecosystem approach.
References
Adams, N.J., 1987. Foraging range of king penguins Aptenodytes patagonicus during summer at Marion Island. J. Zool. [London], 212(3), 475–482.
Adams, N.J. and M.-P. Wilson, 1987. Foraging parameters of gentoo penguins Pygoscelis papua at Marion Island. Polar Biol., 7(1), 51–56.
Adams, N., C. Brown and N. Klages, 1985. Comparison of the diet of four species of penguins breeding on Marion Island, southern Indian Ocean. [Abstract.] S. Afr. J. Sci.,
81, 700. Bester, M.N. and G.J.G. Hofmeyr, 2005. Numbers of ele-
phant seals at Prince Edward Island, Southern Ocean.
S. Afr. J. Wildlife Res., 35(1), 85–88. Brandão, A., D.S. Butterworth, B.P. Watkins and D.G.M.
Miller, 2002. A first attempt at an assessment of the Patagonian toothfish (Dissostichus eleginoides) resource in the Prince Edward Islands EEZ. CCAMLR Sci., 9,
11–32. Brandão, A. and D.S. Butterworth, 2009. A proposed man-
agement procedure for the toothfish (Dissostichus eleginoides) resource in the Prince Edward Islands vicinity. CCAMLR Sci., 16, July, 33–69.
Brown, C.R., 1987. Traveling speed and foraging range of macaroni and rockhopper penguins at Marion Island. J. Field Ornithol., 58(2), 118–125.
CCAMLR, 2010. Appendix R. Fishery Report: Dissostichus eleginoides Prince Edward Islands South African EEZ (Subareas 58.6 and 58.7). Fishery reports 2010. Hobart, Tasmania, Commission for the Conservation of Antarctic Marine Living Resources (CCAMLR), digital media. (www.ccamlr.org/en/system/files/appR_0.pdf).
CCAMLR, 2011. Appendix L. Fishery Report: Dissostichus eleginoides Prince Edward Islands South African EEZ (Subareas 58.6 and 58.7) Fishery reports 2011. Hobart, Tasmania, Commission for the Conservation of Antarctic Marine Living Resources (CCAMLR), digital media. (Report Series 8.)
(www.ccamlr.org/en/system/files/appL.pdf).
Crawford, R.J.M. and 11 others, 2003a. Population and breeding of the gentoo penguin Pygoscelis papua at Marion Island, 1994/95–2002/03. Afr. J. Mar. Sci., 25(1),
463–474. Crawford, R.J.M., J. Cooper and B.M. Dyer, 2003b. Popula-
tion of the macaroni penguin Eudyptes chrysolophus at Marion Island, 1994/95–2002/03, with information on breeding and diet. Afr. J. Mar. Sci., 25(1), 475–486.
Crawford, R.J.M. and 8 others, 2003c. Decrease in numbers of the eastern rockhopper penguin Eudyptes chrysocome filholi at Marion Island, 1994/95–2002/03. Afr. J. Mar. Sci., 25(1), 487–498.
Hofmeyr, G.J.G., M.N. Bester, A.B. Makhado and P.A. Pistorius, 2006. Population changes in Subantarctic and Antarctic fur seals at Marion Island: research. S. Afr. J. Wildlife Res., 36(1), 55–68.
Nel, D.C., P.G. Ryan, R.J. Crawford, J. Cooper and O.A.
Huyser, 2002a. Population trends of albatrosses and petrels at sub-Antarctic Marion Island. Polar Biol., 25(2),
81–89. Nel, D.C. and 6 others, 2002b. Foraging interactions
between wandering albatrosses Diomedea exulans breeding on Marion Island and long-line fisheries in the southern Indian Ocean. Ibis, 144(3), E141–E154.
Nel, D.C., P.G. Ryan and B.P. Watkins, 2002c. Seabird mortality in the Patagonian toothfish longline fishery around the Prince Edward Islands, 1996–2000. Ant. Sci., 14(2),
151–161.
Leigh Gurney (lgurney@eos.ubc.ca), a graduate of the University of Cape Town and Rhodes University, is a Ph.D. student in Biological and Fisheries Oceanography with the Department of Earth and Ocean Sciences, University of British Columbia. Evgeny Pakhomov (epakhomov@eos.ubc.ca), a graduate of the Shirshov Institute of Oceanology, Moscow, and an Adjunct Professor with the Department of Zoology, Faculty of Science and Agriculture, University of Fort Hare, Alice, South Africa, is an Associate Professor in Biological and Fisheries Oceanography with the Department of Earth and Ocean Sciences, University of British Columbia.
Canada Research Chair for Christian Haas
Christian Haas is the new Canada Research Chair in Arctic Sea Ice Geophysics at York University. His research in the Arctic and Antarctic, satellite remote sensing and numerical modeling has contributed unique information on sea ice thickness. He is a member of the ASPeCt (Antarctic Sea Ice Processes and Climate) group and coordinator of the International Programme for Antarctic Buoys (IPAB).
Ionospheric GPS Scintillation Monitoring in the Arctic and Antarctic
P. Prikryl and P. T. Jayachandran
Global positioning system (GPS) receivers have become indispensable navigation tools in aviation, seafaring and even when driving on well-signed roads. Before reaching these navigation tools, the satellite radio signal has to travel through many layers of the Earth’s atmosphere. Ionospheric and tropospheric delays must be accounted for in order to obtain accurate positions. Another mportant atmospheric/ionospheric effect on the transionospheric signal is scintillation — rapid fluctuations of amplitude and phase of the trans-ionospheric radio signal caused by irregularities in the electron density of the ionosphere. Intense scintillation causes a GPS receiver to lose lock on a satellite signal, thus making signal tracking difficult. The polar and auroral ionospheres are directly affected by solar storms and space weather that pose a risk to GPS technology. With climate change imminent, the Arctic will be opening up to more human activity, such as increased exploitation of natural resources and commercial developments, which will be increasingly dependent on the Global Navigational Satellite System (GNSS).

Figure 1 (a) The Arctic (CHAIN) and (b) Antarctic GISTM arrays. The corrected geomagnetic (CGM) latitudes 70° and 80° are superposed over the geographic grid.
The Canadian High Arctic Ionospheric Network (CHAIN, http://chain.physics.unb.ca) — an array of ten GPS receivers and six Canadian Advanced Digital Ionosondes — is operated by the University of New Brunswick (Jayachandran and others, 2009) to study the high-latitude ionosphere (Fig. 1a). Figure 2 shows one of the stations located in Qikiqtarjuaq, Nunavut. The GPS receiver provides ionospheric total electron content (TEC) from GPS L1 and L2 signals and computes the amplitude and phase scintillation indices from high-rate (50 Hz) power and phase samples of the GPS L1 signal. The CHAIN array of GPS Ionospheric Scintillation and TEC Monitors (GISTMs) complements similar arrays in northern Europe, including Svalbard (not shown in Fig. 1a), and Antarctica (De Franceschi and others, 2006) (Fig. 1b). Scientific collaboration among nine countries has been supported by the Scientific Committee on Antarctic Research (SCAR) Action Group “GPS for Weather and Space Weather Forecasting” (www.gwswf. scar.org). The aim is to develop ionospheric imaging capability and to share GNSS data in polar research and applications for weather and space weather modelling and forecasting.

The monitoring of L-band scintillation and TEC in both hemispheres offers new opportunities to undertake inter-hemispheric studies of scintillation and TEC, and to improve understanding of space-weather impact on the polar and auroral ionospheres. These in turn affect the operation of GNSS-based communications and navigation systems. Aiming at a capability of forecasting GPS scintillation caused by rapid variations in TEC from ionospheric irregularities, the climatology of phase scintillation has been developed for high latitudes in the auroral and polar regions (Spogli and others, 2009; Alfonsi and others, 2011; Prikryl and others, 2011a). Results show that phase scintillation, as a function of magnetic local time (MLT) and geomagnetic latitude, primarily occurs in the night-side auroral oval and the ionospheric cusp, with scintillation regions shifting in latitude in response to varying geomagnetic activity and seasonal variations.
The most intense scintillation occurs when the ionosphere is impacted by solar wind disturbances, either highspeed solar wind from coronal holes or coronal mass ejections (CMEs) sometimes associated with intense solar flares. The solar wind plasma permeated by the interplanetary magnetic field (IMF), interacts with the Earth’s magnetosphere as a whole, and the coupling between the IMF and geomagnetic field manifests itself in the ionosphere in both heres. In general, the Earth’s dipole magnetic field lines connect conjugate locations in the northern and southern hemispheres, but the polar ionospheres are partly threaded by magnetic field lines directly connected to the solar wind. This magnetic topology can be very complex and may result in significant asymmetries of the observed ionospheric phenomena including aurorae and ionospheric irregularities that cause scintillation in the northern and southern hemispheres.
The collaboration initiated by the SCAR Action Group led to a study of an inter-hemispheric comparison of phase scintillation caused by ionospheric disturbances during the first significant geomagnetic storm of the new solar cycle 24 on 5–6 April 2010 (Prikryl and others, 2011b). This storm was caused by an interplanetary CME of a magnetic cloud (MC) type that was embedded in the high-speed solar wind from a coronal hole, thus increasing the impact on the ionosphere. The arrival of the upstream shock, associated with a solar-wind pressure pulse and southward IMF that caused a substorm onset, produced comparable, but asymmetric, phase scintillation occurrences in the northern and southern hemispheres (Fig. 3). In the polar cap, a strong asymmetry was observed when the IMF was southward and patches of ionization were expected to convect from dayside to nightside in the polar ionosphere. The asymmetry can be explained by the seasonal variation in the occurrence of patches, which peaks in the southern polar cap while being near its lowest in the northern polar cap in April. Ionospheric scintillation-causing irregularities can also be studied with satellites either in situ or using on-board radio instruments including GPS receivers. An example is a small satellite mission funded by the Canadian Space Agency. The Cascade Smallsat and Ionospheric Polar Explorer (CASSIOPE) Enhanced Polar Outflow Probe (e-POP) (Yau and others, 2006) is scheduled to be launched on a high-inclination orbit in 2013. It will collect a variety of ionospheric data sampled at a high rate in both hemispheres. In conjunction with ground facilities around the globe, the e-POP mission will provide a new perspective to the study of radio-wave propagation in the F-region and topside ionosphere. In addition to ionospheric effects, tropospheric delay, which is composed of hydrostatic and wet delays, also affects the GPS/GNSS positioning accuracy. These delays can be estimated using troposphere mapping functions based on weather forecast models, interpolated for a given GNSS station, and can be applied in Precise Point Positioning (PPP) processing (Zumberge and others, 1997; Kouba and Héroux, 2001). Conversely, mapping functions can be used in the retrieval of total precipitable water vapor (PWV) that is valuable as an input to weather forecasting (Wolfe and Gutman, 2000) and atmospheric sensing in remote areas, which may be crucial for short-term forecasts as well as climate-change studies (Vey and others, 2010; Thomas and others, 2011).
Presently, CHAIN is being expanded by adding 15 more GPS stations in the Canadian Arctic and international colleagues have been installing more GPS scintillation receivers in Antarctica, enhancing ionospheric imaging capabilities in both hemispheres. The SCAR Action Group was transformed into an Expert Group “GNSS Research and Application for Polar Environment (GRAPE)” at the SCAR meeting in Portland, Oregon. These activities portend growing international collaboration in Arctic–Antarctic atmospheric research using GNSS.


Figure 2: The CHAIN site in Qikiqtarjuaq, Nunavut, Canada, which has its “conjugate” counterpart at South Pole station in Antarctica.


Figure 3: Phase scintillation occurrence as a function of MLT and CGM latitude during a geomagnetic storm caused by a CME in April 5–6, 2010. Superposed (white line) is the Feldstein statistical auroral oval for IQ = 5 (Feldstein and Starkov, 1967; Holzworth and Meng, 1975). (a) Northern hemisphere; (b) southern hemisphere.
References
Alfonsi, L. and 6 others, 2011. Bipolar climatology of GPS
ionospheric scintillation at solar minimum. Radio Sci.,
46(3), RS0D05 (10.1029/2010RS004571).
De Franceschi, G., L. Alfonsi and V. Romano, 2006.
ISACCO: an Italian project to monitor the high latitude ionosphere by means of GPS receivers. GPS Solutions, 10(4),
263–267. Feldstein, Y.I. and G.V. Starkov, 1967. Dynamics of auroral belt
and polar geomagnetic disturbances. Planet. Space Sci.,
15(2), 209–230.
Holzworth, R.H. and C.-I. Meng, 1975. Mathematical representation of the auroral oval. Geophys. Res. Lett., 2(9),
377–380. Jayachandran, P.T. and 12 others,. 2009. Canadian High Arctic
Ionospheric Network (CHAIN). Radio Sci., 44, Special Section, RS0A03 (10.1029/2008RS004046, printed 45(1),
2010). Kouba, J. and P. Héroux, 2001. Precise point positioning using
IGS orbit and clock products. GPS Solutions, 5(2), 12–28. Prikryl, P., P.T. Jayachandran, S.C. Mushini and R. Chadwick,
2011a. Climatology of GPS phase scintillation and HF radar backscatter for the high-latitude ionosphere under solar minimum conditions. Ann. Geophys. (ANGEO), 29(2),
377–392. Prikryl, P. and 18 others, 2011b. Interhemispheric comparison
of GPS phase scintillation at high latitudes during the magnetic-cloud-induced geomagnetic storm of 5–7 April 2010. Ann. Geophys. (ANGEO), 29(12), 2287–2304.
Spogli, L., L. Alfonsi, G. De Franceschi, V. Romano, M.H.O.
Aquino and A. Dodson, 2009. Climatology of GPS ionospheric scintillations over high and mid-latitude European regions. Ann. Geophys. (ANGEO), 27(9), 3429–3437.
Thomas, I.D., M.A. King, P.J. Clarke and N.T. Penna, 2011. Precipitable water vapor estimates from homogeneously reprocessed GPS data: an intertechnique comparison in Antarctica. J. Geophys. Res., 116(D4), D04107 (10.1029/2010JD013889).
Vey, S., R. Dietrich, A. Rülke, M. Fritsche, P. Steigenberger and M. Rothacher, 2010. Validation of precipitable water vapor within the NCEP/DOE reanalysis using
global GPS observations from one decade. J. Climate, 23(7),
1675–1695.
Wolfe, D.E. and S.I. Gutman, 2000. Developing an operational, surface-based, GPS, water vapor observing system for NOAA: network design and results. J. Atmos. Oceanic Technol., 17(4), 426–440.
Yau, A.W., H.G. James and W. Liu, 2006. The Canadian Enhanced Polar Outflow Probe (e-POP) mission in ILWS. Adv. Space Res., 38(8), 1870–1877.
Zumberge, J.F., M.B. Heflin, D.C. Jefferson, M.M. Watkinsand F.H. Webb, 1997. Precise point positioning for the efficient and robust analysis of GPS data from large networks. J. Geophys. Res., 102(B3), 5005–5017.
Paul Prikryl (paul.prikryl@crc.gc.ca) is a Research Scientist at the Communications Research Centre Canada in Ottawa. Thayyil Jayachandran (jaya@unb.ca) is a Professor at the Physics Department of the University of New Brunswick in Fredericton. Both are participants in GRAPE.
XXXII SCAR Meetings and Open Science Conference, Portland, Oregon, U.S.A., 13–25 July, 2012
The 32nd biennial SCAR meeting was held in Portland, Oregon, U.S.A., from 13 to 25 July 2012. The business meetings, which took place during the first five days, were an opportunity for members of the three Standing Scientific Groups (SSG) and the two Standing Committees (SG) to review progress and confirm objectives for the next two years and for expert groups, such as that on Ice Sheet Mass Balance and Sea Level (ISMASS), to hold meetings and workshops. Kathy Conlan (Canadian Museum of Nature) completed her term as Chief Officer of Life Sciences (SSG-LS) and was successful in launching two new programs. Simon Ommanney (Secretary of CCAR) attended meetings of the SG on Antarctic Geographic Information (SG-AGI), the SG on Antarctic Data Management (SG-ADM), and part of the meeting of the SSG on Physical Sciences (SSG-PS) that was also attended by Tom James (Geological Survey of Canada). Gail Fondahl (UNBC) participated in activities of the Joint IASC/SCAR Bipolar Advisory Group on Science Cooperation (BipAG II). The SCAR meeting was also an opportunity for our international colleagues to meet the new Executive Director of the Canadian Polar Commission, David Scott, who is Canada’s Chief Delegate to SCAR. An Open Science Conference on Antarctic Science and Policy Advice in a Changing World took place from 16–19 July. It was good to see two Canadian companies that support a number of national Antarctic programs as sponsors of the meeting — Canada Goose Inc. and Kenn Borek Air Ltd. Considering the proximity of the venue to Canada, Canadian attendance was quite limited, likely affected by the earlier International Polar Year (IPY) meeting held in April in Montréal. A list of presentations by Canada-affiliated authors (in bold letters) follows. The delegates met from 23–25 July, in plenary and in two groups, to map out the future direction of SCAR, approve new programs and consider reports and recommendations from the SSGs and SGs. A report on the meeting of the science groups follows.
Open Science Conference Presentations
Bers, A.V., F. Momo, I. Schloss, D. Abele and M. Braun.
Analysis of trends and sudden changes in environmental long-term data from King George Island (Antarctica): relationships between global climatic oscillations and local system response.
Cilliers, P. and 7 others (including P. Prikryl). Space weather impact on the upper atmosphere over the South Atlantic magnetic anomaly.
Crump, J. and I. Kelman. Many strong voices: climate change and resilience in the Arctic and small island developing states.
Dayton, P., S. Kim, K. Conlan and S. Jarrell. Episodic growth of sponges on artificial structures in McMurdo Sound, Antarctica.
Fleming, A., H. Corr, S. Achal, J. McFee and K. Hughes.
Combining airborne hyperspectral data and satellite imagery for monitoring of the Antarctic Peninsula. Fondahl, G. and J. Larsen. Assessing change in the Arctic:
the human development report and the Arctic Social
Indicators project.
Gorman, K.B., K.E. Ruck, T.D. Williams and W.R. Fraser.
Trophic interactions and variation in reproductive performance within a community of Antarctic penguins (genus Pygoscelis).
Gorman, K.B., T.D. Williams and W.R. Fraser. Stress hormone modulation of reproductive performance within a community of Antarctic penguins (genus Pygoscelis).
Haupt, T., E. Marais, B. Sinclair and S. Chown. Metabolic costs in the face of climate change: a keystone species on sub-Antarctic Marion Island.
Hoppe, C.J.M., C. Hassler, C.D. Payne, P. Tortell, B. Rost and S. Trimborn. Combined effects of ocean acidification and iron availability on Southern Ocean phytoplankton communities.
Hosie, G. and 10 others (including S.D. Batten). Initiation of a Global Alliance of Continuous Plankton Recorder Surveys (GACS).
Ibarguchi, G., P. Convey and V.L. Friesen. Out of Antarctica, into the cold: overlooked diversification of alpine, polar, and temperate lineages around the world.
King, M. and 6 others (including G. Milne). The contribution of the Antarctic Ice Sheet to present-day sea-level change.
Kruse, S., E. Pakhomov, B. Hunt and U. Bathmann. Seasonal and latitudinal variability of the food web structure in the Lazarev Sea.
Murray, A. and 7 others (including C. Lovejoy). Comparisons between bacterioplankton from the Southern Ocean and Arctic Ocean provide perspective on distinctiveness of polar marine systems.
Nelson, T.M., M.V. Brown, T.L. Rogers and J.M. Fairbrother. Investigating Escherichia coli pathotypes and antimicrobial resistance patterns in wild southern elephant seals, Antarctica.
Polito, M., R. Brasso, W. Trivelpiece, W. Patterson and S.
Emslie. Generalist and specialist foraging strategies influence mercury (Hg) exposure in sympatrically breeding Pygoscelis penguins.
Prikryl, P. and 15 others (including R. Ghoddousi-Fard, P.T.
Jayachandran, D.W. Danskin, E.L. Spanswick and E.F. Donovan). Interhemispheric comparison of GPS phase scintillation and auroral emission observed from the ground and DMSP satellite.
Prikryl, P., P.T. Jayachandran, S.C. Mushini and I.G.
Richardson. Probabilistic forecasting of high-latitude GPS
phase scintillation. Quartino, L. and 14 others (including I. Schloss). ESF-
IMCOAST: an integrated multi-disciplinary study of coastal climate change effects in Western Antarctica.
Schwartz, M.J., G.L. Manney, W.H. Daffer, M.I. Hegglin and K.A. Walker. UTLS satellite measurements of chemical trace gases in the context of multiple tropopauses and upper-tropospheric jets.
Suedfeld, P., G.D. Steel and J. Brcic. Personality and the positive psychological impact of polar sojourns.
Vincent, W. and C. Lovejoy. Antarctic analogues: microbial ecosystems and comparative biodiversity in the Canadian High Arctic.
XXXII SCAR Meeting
Delegate Committee on Scientific Affairs
C. Simon L. Ommanney
The Scientific Committee on Antarctic Research, through its three Standing Scientific Groups, sponsors a number of Scientific Research Programmes (SRPs). These are established for a finite period of time so periodically there is a period of reflection when the science programs are reassessed and refocused to ensure they adequately address the emerging scientific concerns. The SCAR meeting in Portland was such a time, when the mandate of three of the SRPs was expiring and delegates were asked to consider and approve five new programs that Antarctic scientists were recommending. Drawing to a close were Antarctic Climate Evolution (ACE), Evolution and Biodiversity in the Antarctic (EBA) and Antarctica and the Global Climate System (AGCS).
Scientists under the umbrella of ACE have been studying the climate and glacial history of Antarctica through palaeoclimate investigations and ice-sheet modeling, looking at both the recent and distant geologic past, including times when the Earth was several degrees warmer than today. There were obviously close links between this program and AGCS, which was investigating the nature of atmospheric and oceanic linkages between the Antarctic climate and the rest of the Earth system, and their mechanisms. It made use of data from deep and shallow ice cores, satellite data, and the output of global and regional GCMs. EBA brought together a number of pre-existing SCAR programs aimed at understanding the evolution and diversity of life in the Antarctic. It was considering influences on the properties and dynamics of present-day Antarctic and Southern Ocean ecosystems and how the systems might respond to current and future environmental change. In all cases the timeframe for answering the questions posed was inadequate, so in the transition to new programs many of the remaining outstanding, unanswered questions will be carried over. When considering a successor to EBA, it was recognized that the community trying to deal with the questions was really too large for effective communication. It made sense to divide the problems in two. The first will deal with the State of the Antarctic Ecosystem (AntEco), focusing on patterns of biodiversity across terrestrial, limnological, glacial and marine environments within the Antarctic, subAntarctic and Southern Ocean regions. Its primary purpose is to explain the existing biodiversity — how it got there, what it does and what threatens it. The second will deal with Antarctic Thresholds – Ecosystem Resilience and Adaptation (AnT-ERA), defining the thresholds of current biological systems; thereby determining their resistance and resilience to change and thence possible tipping points.
The three other new programs build on earlier ones. Antarctic Climate Change in the 21st Century (AntClim21) hopes to be able to predict key elements of the Antarctic cryosphere, atmosphere and ocean for the next 20 to 200 years and their responses to natural and anthropogenic forcings. Past Antarctic Ice Sheet Dynamics (PAIS) will look at how the Antarctic ice sheet responded to previous warm conditions, with a focus on particularly vulnerable areas on the east and west margins. Whereas Solid Earth Responses and influences on Cryospheric Evolution (SERCE) is considering present conditions, seeking to improve our understanding of the interactions between the solid Earth and the cryosphere, including glacial isostatic adjustment (GIA) and ice-mass change, and the influence of parameters such as heat flow and sediments on ice-sheet dynamics.
Those with relevant experience to contribute to these new programs might consider contacting the principal investigators to see how they might become involved (www.scar.org).
Renewal was also seen in the SSGs with the appoint ment of a new Chief Officer (CO), Deputy Chief Officer (DCO) and Secretary (S): for Life Sciences – Graham Hosie (CO), Marc Shepanek (DCO) and Jan Ropert-Coudert (S); for Geosciences – Berry Lyons (CO), Jesus Galindo-Zaldivar (DCO) and Naresh Pant (S); and for Physical Sciences – David Bromwich (CO), Maurizio Candidi (DCO) and SteveColdwell (S). A more detailed report on the meeting hasbeen published in the SCAR Bulletin, No.182, September 2012 (www.scar.org/publications/bulletins/ Bulletin183.pdf).
Simon Ommanney (simon.ommanney@ns.sympatico.ca) is Canada’s Alternate Delegate to SCAR and Secretary of the Canadian Committee on Antarctic Research.
Origin, Stability and Habitability of Ice-Bearing Permafrost in University Valley, McMurdo Dry Valleys, Antarctica:
Analogue for Ground Ice on Mars
Denis Lacelle, Wayne Pollard, Lyle Whyte, Alfonso Davila, Dale Andersen, Regina DeWitt, Jackie Goordial, Jennifer Heldmann, Margarita Marinova, Kris Zacny and Christopher McKay
Now that the Phoenix Mars Lander has confirmed the presence of water on Mars (Smith and others, 2009), the next step is to explore the Martian subsurface environment. To help define the technological and scientific requirements of such a mission, Canadian permafrost scientists, Denis Lacelle (University of Ottawa) and Wayne Pollard (McGill), and geomicrobiologist Lyle Whyte (McGill), are working with NASA and SETI scientists, and Honeybee engineers, in a multiyear (2009–2013) NASA-supported research project called IceBite (Fig. 1). The main objective of the project is to develop a flight-ready system capable of drilling and sampling the Martian ice-cemented regolith to 1 m depth (IceBreaker Life mission; McKay, In review), and to enhance our understanding of the physical, chemical and biological interactions that take place in dry and ice-bearing permafrost in the upper Dry Valleys of Antarctica. The extreme cold and aridity of the McMurdo Dry Valleys (MDV, widely known as the coldest, driest and most Mars-like place on Earth) is being used as a terrestrial analogue to understand how frozen landscapes, and possibly even life, may have developed on Mars. In addition, the project will enhance our understanding of the dynamics of water vapour exchanges between different ice reservoirs (polar ice cap and ground ice) and the atmosphere in an ultraxerous climate zone. The major science objectives in the upper MDV are to:
1) determine the origin and age of ice-bearing sediments;
2) assess the stability of near-surface ground ice under current and past climatic conditions;
3) assess the habitability and presence of microbes and complex biomolecules in ice-bearing permafrost;
4) develop a model of ground-ice emplacement (condensation, adsorption and effects of deliquescent salts) under Mars-like conditions. Overall, the project will help us better understand how ice-bearing permafrost of vapour-diffusion origin behaves on Earth and Mars.

Figure 1: IceBite team in University Valley, December 2009. From left to right: Margarita Marinova, Alfonso Davila, Jennifer Heldmann, Dale Andersen, Christopher McKay, Wayne Pollard and Denis Lacelle
The MDV are the largest ice-free region in Antarctica. They consist of a series of east–west trending ice-free valleys located between the East Antarctic ice sheet and the Ross Sea. The valleys are ~80 km long and up to 15 km wide, with elevations ranging from sea level near the Ross Sea to ~2000 m a.s.l. at the junction with the Polar Plateau. Climatically, the MDV are all cold and arid environments, part of the polar desert climate regime. However, an elevation-controlled climate gradient exists (Doran and others, 2002; Marchant and Head, 2007). The lower valleys (<400 m a.s.l.) are the warmest and wettest, whereas the upper valleys (>1000 m a.s.l.) are the coldest and driest, with summer air temperatures nearly always below 0°C. The upper elevations owe this characteristic to the Transantarctic Mountains that block glacial ice from moving to the coast and to the strong katabatic winds that originate high on the East Antarctic ice sheet. These winds cause a greater amount of evaporation relative to the low amount of precipitation received, resulting in a water deficit.
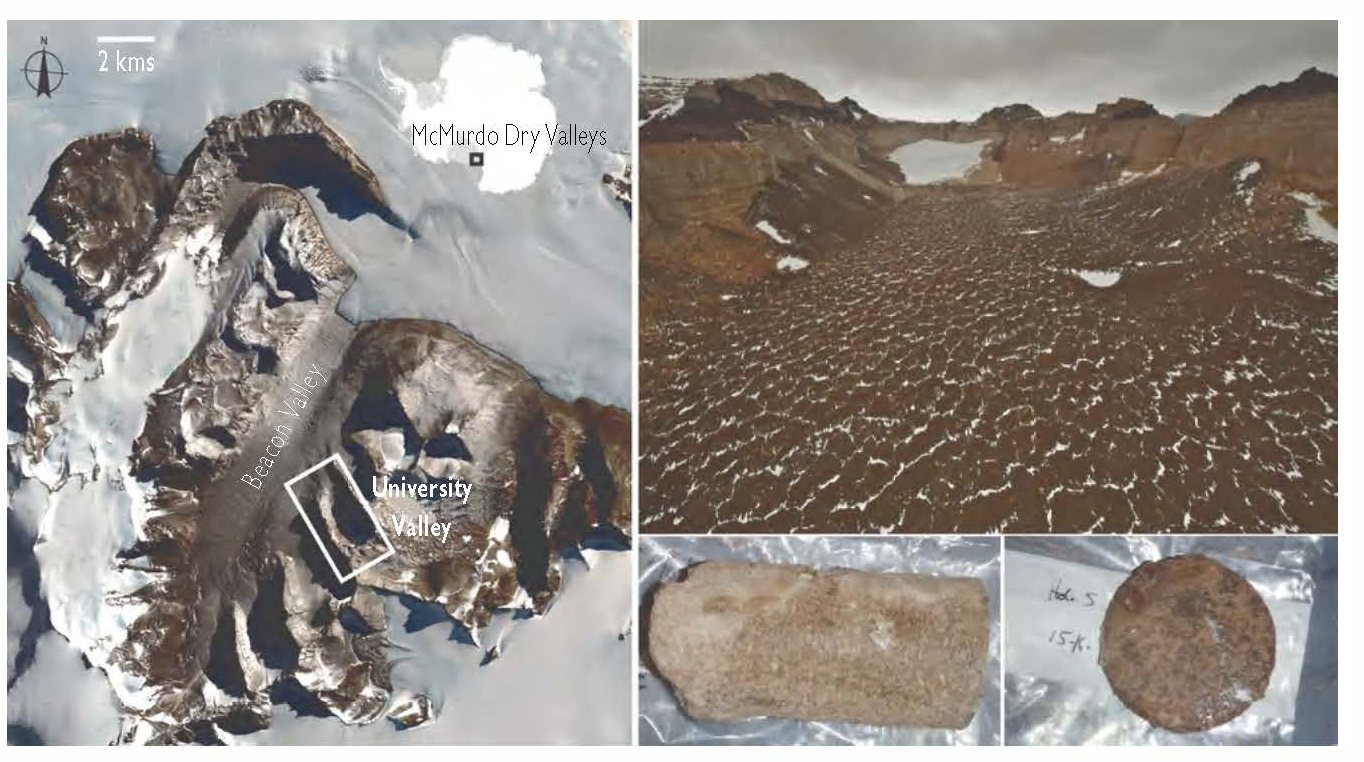
Figure 2: Upper valleys of MDV (left) showing University Valley. Aerial view of University Valley with small ice-patch at the head of the valley (top right). Photographs of ice-cemented permafrost cores (bottom right). Photo: NGA Commercial Imagery Program ©DigitalGlobe Inc
The study site is in University Valley (77°52´S; 160°40´E; 1700 m a.s.l.), one of the upper valleys of the Quartermain Mountains in the MDV (Fig. 2). It is a 4 km long, 1.5 km wide, NW-facing hanging glacial valley some ~450 m above the floor of Beacon Valley. A small permanent ice-patch partially covers the head of the valley and permanent snow patches are also found scattered on the valley floor. Polygonal ground dominates the floor of the valley, which suggests that subsurface ice is extensive. In December 2009, an automated Campbell weather station, equipped with air and soil temperature, relative humidity, solar radiation and wind-speed sensors, was installed in the valley. Mean annual air temperature and relative humidity, from December 2009 to December 2010, was –24.3°C and 48%, respectively. Even though hourly summer air temperatures were always below freezing (maximum recorded temperature: –2.8°C), solar heating raised near-surface soil temperatures above 0°C for a few weeks, allowing the 0°C isotherm to penetrate as much as 10 cm into the soil at the meteorological station site. In University Valley, McKay (2009) observed that the depth to the ice-table increases as a function of distance from the glacier; being <1 cm at the foot of the glacier and increasing to >35 cm 500 m from it. In 2010, hundreds of additional random measurements of ice-table depths across the valley floor supported McKay’s observations. We hypothesize that the ice-table depth varies due to snow recurrence intervals and local topographic effects. The topography of the narrow valley largely controls the amount of solar radiation received at the surface, which influences (near-) surface energy-exchange processes, including snow and ground-ice stability.
To determine the age of sediments in University Valley, five OSL (optically stimulated luminescence) ages were obtained from a 95 cm long core through ice-bearing sediments near the permanent ice-patch where the ice-table depth was 1 cm. The OSL ages in the core increased from 17 ± 3 Ka at 2–7 cm depth to 149.7 ± 16.2 Ka at 92–95 cm below the surface. The increase in OSL ages with depth indicates that the valley floor is an accretionary surface with the sediments being derived largely from the physical and biological weathering of sandstone and dolerite rocks from the surrounding valley walls. Ice-bearing permafrost commonly develops in the pore spaces of coarse-grained sediments (i.e., sand-sized), and results from the in situ freezing of moisture present in the soil, surface melt-water percolating into the soil, or vapour diffusing in the soil from the atmosphere. Even though icecemented permafrost occupies approx. 55% of the upper1 m in the landscape of the MDV (Bockheim and others, 2007), little is known regarding its nature, origin and stability. Studies conducted at lower-elevation valleys, where summer air/soil temperatures exceed the freezing point, suggest that ice-bearing soils form from water supplied to the subsurface by snow and glacier melt (i.e., Dickinson and Rosen, 2003; Hagedorn and others, 2010). However, in the upper valleys, where summer air/soil temperatures rarely exceed 0°C, liquid water is much rarer and transient, yet ice-bearing permafrost is extensive. Here, cryostratigraphic observations, combined with isotopic and geochemical analyses of ice-bearing permafrost suggest that the ice-bearing permafrost forms mainly from the diffusion of water vapour in the sediments, both condensation and adsorption (Lacelle and others, in review). However, given the high concentration of ice in parts of our core samples (up to 84% volumetric ice content), a process is needed to produce ground ice by vapour diffusion over that allowed by the pore space of the sandy-clay loam sediments. Thermally driven contraction–expansion of the frozen sediments may be responsible for the excess ice content measured in the core. Applying the Fisher (2005) numerical model of thermal contraction and vapour-diffusion with local climate data fits well with the measured volumetric ice content within the OSL-imposed time frame (Lacelle and others, in review).
The stability of ice-bearing permafrost was assessed from two bodies of massive ground ice discovered in University Valley (Pollard and others, 2012). The first, adjacent to the perennial ice-patch at the head of University Valley, is interpreted as glacier ice buried by a rockslide. The second body of massive ice is at the mouth of the valley in an area of polygonal moraine. GPR surveys revealed that the first ice body is continuous and extends about 5–6 m beneath a thin boulder-rich debris layer. To determine if the buried massive ground ice bodies are sublimating or in equilibrium with current climatic conditions, the ice was cored and analyzed for O–H isotopes at high vertical resolution. The measurements showed an upward convex d18O (dD) profile towards the ice–soil interface, suggesting that the ice is sublimating.

Figure 3: Schematic diagram of science activities in University Valley, MDV. Major science questions: 1) What controls the ice-table depth? Approach: investigate soil–atmosphere interactions; 2) What is the origin, age and stability of ice-bearing permafrost? Approach: combine cryostratigraphic and isotope-geochemistry measurements with OSL dating; 3) Are the microbes alive and functioning ecosystems or are they frozen in time? Approach: perform culture-dependant and independent analysis and determine if the environment is physically and chemically favourable to microbial growth.
Lacelle and others (2011) developed a sublimation and molecular diffusion model using d18O measurements in ground ice to estimate sublimation rates in a cold-dry environment and the sublimation-rate value (3.0 to 5.0 10–5 mm yr–1) was similar to the one obtained by Ng and others (2005) from buried massive ground ice in Beacon Valley.
The MDV are one of the harshest environments on Earth for life, characterized by extreme cold, arid, and nutrient-poor soils. The potential for them to support life at subzero temperatures is being investigated through a combination of culture-dependent and culture-independent approaches as part of this multidisciplinary project. One of the primary objectives of the microbiology component is to examine whether microorganisms found in the MDV soils are capable of metabolic activity at temperatures similar to in situ conditions, or if they are simply dormant microbes or microbes preserved in the ice-bearing permafrost. In order to address this, a highly sensitive radiorespiration assay (Steven and others, 2007), which measures microbial metabolic activity at subzero temperatures, is being carried out in a long-term (1 year) growth experiment at McGill Uni versity. The measured respiration rates are revealing an aerobic heterotrophic community that is significantly lower in activity than those found previously in other cryoenvironments, such as the Canadian High Arctic. Additionally, a microbial community that is largely recalcitrant to culturing is being found in the MDV soils. Bacterial and fungal isolates are being cultured at cold and subzero temperatures, and those which show interesting cold adaptations will be subjected to state-of-the art genome sequencing. Furthermore, molecular methods are being used to examine the diversity and community composition of the indigenous microorganisms based on DNA extracted directly from sediments, and a metagenome project will determine the functional potential of these communities. Such analyses will contribute significantly to our understanding of microbial diversity, ecology, adaptation, and evolution in this extreme cryoenvironment and will provide new insights into the molecular adaptations of microbial life at subzero temperatures.
Ground ice on Mars is present as ice-bearing ground underlying a layer of dry soil (Smith and others, 2009). The depth to, and stability of, ground ice on Mars is determined by vapour exchange with the atmosphere (Mellon and others, 2009). University Valley is one of the few places on Earth where Mars-like permafrost is found; that is, a layer of dry cryotic sediments overlying ice-bearing sediments. In areas with no active layer, the ice-cemented permafrost in University Valley originates mainly by vapour-diffusion (condensation and adsorption), again a process similar to that on Mars. The excess ground ice accumulates by vapour diffusion; thermal contraction of the sediments allows for ice to build up above the pore spaces. The upper surface of ground ice loses mass by sublimation at a rate dependent on air temperature, humidity and the thermophysical properties of the overlying lag. The studies in University Valley are yielding novel insights into the dynamics of condensation, adsorption and sublimation of water vapour in colddry environments, and our findings are enhancing our understanding of the Martian water cycle, between the various water reservoirs.
References
Bockheim, J.G., I.B. Campbell and M. McLeod, 2007. Permafrost distribution and active-layer depths in the McMurdo Dry Valleys, Antarctica. Permafrost Periglac. Process., 18(3), 217–227.
Dickinson, W.W. and M.R. Rosen, 2003. Antarctic permafrost: an analogue for water and diagenetic minerals on Mars. Geology [Boulder], 31(3), 199–202.
Doran, P.T. and 6 others, 2002. Valley floor climate observations from the McMurdo dry valleys, Antarctica,
1986–2000. J. Geophys. Res., 107(D24), 4772. (10.1029/2001JD002045.)
Fisher, D.A., 2005. A process to make massive ice in the Martian regolith using long-term diffusion and thermal cracking. Icarus, 179(2), 387–397.
Hagedorn, B., R.S. Sletten, B. Hallet, D.F. McTigue and E.J.
Steig, 2010. Ground ice recharge via brine transport in frozen soils of Victoria Valley, Antarctica: Insights from modeling d18O and dD profiles. Geochim. Cosmochim. Acta, 74(2), 435–448.
Lacelle, D. and 8 others, in review. Excess ground ice of condensation-diffusion origin in University Valley, Dry Valleys of Antarctica: evidence from isotope geochemistry and numerical modeling. Geochim. Cosmochim. Acta.
Lacelle, D. and 6 others, 2011. Stability of massive ground ice bodies in University Valley, McMurdo Dry Valleys of Antarctica: using stable O–H isotope as tracers of sublimation in hyper-arid regions. Earth Planet. Sci. Lett.,
301(1–2), 403–411. Marchant, D.R. and J.W. Head, III, 2007. Antarctic Dry Val-
leys: microclimate zonation, variable geomorphic processes, and implications for assessing climate change on Mars. Icarus, 192(1), 187–222.
McKay, C.P., 2009. Snow recurrence sets the depth of dry permafrost at high elevations in the McMurdo Dry Valleys of Antarctica. Ant. Sci., 21(1), 89–94.
McKay, C.P. and 17 others, in review. The IceBreaker Life mission to Mars: a search for biomolecule evidence for life. Astrobiology.
Mellon, M.T. and 14 others. 2009. Ground ice at the Phoenix landing site: stability state and origin. J. Geophys. Res.,
114, Special Section, E00E07. (10.1029/2009JE003417, printed 115(E1), 2010.)
Ng, F., B. Hallet, R.S. Sletten and J.O. Stone, 2005. Fastgrowing till over ancient ice in Beacon Valley, Antarctica. Geology [Boulder], 33(2), 121–124
Pollard, W.H. and 6 others, 2012. Ground ice conditions in University Valley, McMurdo Dry Valleys, Antarctica. In: K.M. Hinkel, ed., Proceedings of the Tenth International Conference on Permafrost, Salekhard, Yamal-Nenets Autonomous District, Russia, June 25–29, 2012, Vol. 1: International Contributions. Salekhard, The Northern Publisher, 305–310.
Smith, P.H. and 35 others, 2009. H2O at the Phoenix landing site. Science, 325(5936), 58–61.
Steven, B., T.D. Niederberger, E.M. Bottos, M.R. Dyen and L.G. Whyte, 2007. Development of a sensitive radiorespiration method for detecting microbial activity at subzero temperatures. J. Microbiol. Meth., 71(3), 275–280.
Denis Lacelle (dlacelle@uottawa.ca) is affiliated with the Department of Geography at the University of Ottawa, Ontario. Wayne Pollard, Lyle Whyte and Jackie Goordial are at McGill University, Montréal, the first in the Department of Geography, the others in the Department of Natural Resource Sciences. United States’ collaborators are: Alfonso Davila and Dale Andersen, with the SETI Institute in Mountain View, CA; Regina DeWitt, with the Department of Physics at Oklahoma State University in Stillwater, OK; Jennifer Heldmann, Margarita Marinova and Christopher McKay all at the NASA Ames Research Center in Moffett Field, CA; and Kris Zacny, with the Honeybee Robotics Spacecraft Mechanisms Corporation in Pasadena, CA.
CCAR/CCRA Members and Advisers
Marianne Douglas, Director (Chair)
Canadian Circumpolar Institute University of Alberta
8625 – 112 Street
Edmonton, Alberta T6G 0H1
Tel: (780) 492-0055
Fax: (780) 492-1153 msdougla@ualberta.ca
Kathy Conlan Canadian Museum of Nature
P.O. Box 3443, Station D
Ottawa, Ontario K1P 6P4
Tel: (613) 364-4063
Fax: (613) 364-4027 kconlan@mus-nature.ca
Thomas S. James
Geological Survey of Canada
Natural Resources Canada
9860 West Saanich Road
P.O. Box 6000
Sidney, British Columbia V8L 4B2
Tel: (250) 363-6403
Fax: (250) 363-6565 tjames@nrcan.gc.ca
Émilien Pelletier
Institut des sciences de la mer de Rimouski (ISMER)
310, allée des Ursulines, C.P. 3300
Rimouski, Québec G5L 3A1
Tel: (418) 723-1986 x 1764
Fax: (418) 724-1842 emilien_pelletier@uqar.qc.ca
Peter L. Pulsifer, Research Assoc.
Geomatics and Cartographic Research
Centre Carleton University, and National Snow and Ice Data Center
449 UCB, University of Colorado Boulder, CO 80309,
USA Tel: (613) 620-7195
Fax: (613) 249-7067 pulsifer@nsidc.org
Fred Roots (Antarctic Adviser CPC) Environment Canada
351 St. Joseph Blvd, 1st Floor
Ottawa, Ontario K1A 0H3
Tel: (819) 997-2393
Fax: (819) 997-5813 fred.roots@ec.gc.ca
Wayne Pollard (Past Chair) Department of Geography McGill University
805 Sherbrooke Street West
Montréal, Quebec H3A 2K6
Tel: (514) 398-4454
Fax: (514) 398-7437 pollard@felix.geog.mcgill.ca
Simon Ommanney (CCAR Secretary)
56 Spinney Road, P.O. Box 730
R.R. #1, Glenwood, Yarmouth County
Nova Scotia B0W 1W0
Tel: (902) 643-2527 simon.ommanney@ns.sympatico.ca
Editor: C. Simon L. Ommanney Please send contributions and correspondence to: C. Simon L. Ommanney
Editor, CARN Newsletter
Address above.
Canadian Polar Commission Suite 1710, 360 Albert Street Ottawa, Ontario K1R 7X7
Tel: (613) 943-8605
Fax: (613) 943-8607 mail@polarcom.gc.ca www.polarcom.gc.ca
All rights reserved
Canadian Polar Commission/ Canadian Antarctic Research Network ISSN 1499 0148
- Date modified: